The Big Bang theory-The principle of the Universe
The Big Bang occurred approximately 13.798 ± 0.037 billion years ago
The Big Bang theory is the prevailing cosmological model that describes the early development of the Universe. According to the theory, the Big Bang occurred approximately 13.798 ± 0.037 billion years ago, which is thus considered the age of the universe. At this time, the Universe was in an extremely hot and dense state and began expanding rapidly. After the initial expansion, the Universe cooled sufficiently to allow energy to be converted into various subatomic particles, including protons, neutrons, and electrons.
The Big Bang theory is the prevailing cosmological model that describes the early development of the Universe. According to the theory, the Big Bang occurred approximately 13.798 ± 0.037 billion years ago, which is thus considered the age of the universe. At this time, the Universe was in an extremely hot and dense state and began expanding rapidly. After the initial expansion, the Universe cooled sufficiently to allow energy to be converted into various subatomic particles, including protons, neutrons, and electrons.
Though simple atomic nuclei formed within the first three minutes after the Big Bang, thousands of years passed before the first electrically neutral atoms formed. The majority of atoms that were produced by the Big Bang are hydrogen, along with helium and traces of lithium. Giant clouds of these primordial elements later coalesced through gravity to form stars and galaxies, and the heavier elements were synthesized either within stars or during supernovae.
The Big Bang is the scientific theory that is most consistent with observations of the past and present states of the universe, and it is widely accepted within the scientific community.
It offers a comprehensive explanation for a broad range of observed phenomena, including the abundance of light elements, the cosmic microwave background, large scale structure, and the Hubble diagram.
The core ideas of the Big Bang—the expansion, the early hot state, the formation of light elements, and the formation of galaxies—are derived from these and other observations.
It offers a comprehensive explanation for a broad range of observed phenomena, including the abundance of light elements, the cosmic microwave background, large scale structure, and the Hubble diagram.
As the distance between galaxies increases today, in the past galaxies were closer together. The consequence of this is that the characteristics of the universe can be calculated in detail back in time to extreme densities and temperatures, while large particle accelerators replicate such conditions, resulting in confirmation and refinement of the details of the Big Bang model.
On the other hand, these accelerators can only probe so far into high energy regimes, and astronomers are prevented from seeing the absolute earliest moments in the universe by various cosmological horizons. The earliest instant of the Big Bang expansion is still an area of open investigation. The Big Bang theory does not provide any explanation for the initial conditions of the universe; rather, it describes and explains the general evolution of the universe going forward from that point on.
On the other hand, these accelerators can only probe so far into high energy regimes, and astronomers are prevented from seeing the absolute earliest moments in the universe by various cosmological horizons. The earliest instant of the Big Bang expansion is still an area of open investigation. The Big Bang theory does not provide any explanation for the initial conditions of the universe; rather, it describes and explains the general evolution of the universe going forward from that point on.
Georges Lemaître first proposed what became the Big Bang theory in what he called his "hypothesis of the primeval atom". Over time, scientists built on his initial ideas to form the modern synthesis. The framework for the Big Bang model relies on Albert Einstein's general relativity and on simplifying assumptions such as homogeneity and isotropy of space. The governing equations were first formulated by Alexander Friedmann and similar solutions were worked on by Willem de Sitter.
In 1929, Edwin Hubble discovered that the distances to far away galaxies were strongly correlated with their redshifts—an idea originally suggested by Lemaître in 1927. Hubble's observation was taken to indicate that all very distant galaxies and clusters have an apparent velocity directly away from our vantage point: the farther away, the higher the apparent velocity, regardless of direction. Assuming that we are not at the center of a giant explosion, the only remaining interpretation is that all observable regions of the universe are receding from each other.
In 1929, Edwin Hubble discovered that the distances to far away galaxies were strongly correlated with their redshifts—an idea originally suggested by Lemaître in 1927. Hubble's observation was taken to indicate that all very distant galaxies and clusters have an apparent velocity directly away from our vantage point: the farther away, the higher the apparent velocity, regardless of direction. Assuming that we are not at the center of a giant explosion, the only remaining interpretation is that all observable regions of the universe are receding from each other.
Two expanding universe theories
A ) - Steady state theory
B ) - Big Bang theory
A ) - Steady state theory
B ) - Big Bang theory
While the scientific community was once divided between supporters of two different expanding universe theories—the Big Bang and the Steady State theory, observational confirmation of the Big Bang scenario came with the discovery of the cosmic microwave background radiation in 1964, and later when its spectrum (i.e., the amount of radiation measured at each wavelength) was found to match that of thermal radiation from a black body. Since then, astrophysicists have incorporated observational and theoretical additions into the Big Bang model, and its parametrization as the Lambda-CDM model serves as the framework for current investigations of theoretical cosmology.
There are generally considered to be three outstanding problems with the Big Bang theory: the horizon problem, the flatness problem, and the magnetic monopole problem. The most common answer to these problems is inflationary theory; however, since this creates new problems, other options have been proposed, such as the Weyl curvature hypothesis.
Problems of the Big Bang theory
A ) -The horizon problem
The horizon problem results from the premise that information cannot travel faster than light. In a Universe of finite age this sets a limit—the particle horizon—on the separation of any two regions of space that are in causal contact. The observed isotropy of the CMB is problematic in this regard: if the Universe had been dominated by radiation or matter at all times up to the epoch of last scattering, the particle horizon at that time would correspond to about 2 degrees on the sky. There would then be no mechanism to cause wider regions to have the same temperature.
B ) - The flatness problem
B ) - The flatness problem
The flatness problem (also known as the oldness problem) is an observational problem associated with a Friedmann–Lemaître–Robertson–Walker metric. The Universe may have positive, negative, or zero spatial curvature depending on its total energy density. Curvature is negative if its density is less than the critical density, positive if greater, and zero at the critical density, in which case space is said to be flat.
The problem is that any small departure from the critical density grows with time, and yet the Universe today remains very close to flat. notes Given that a natural timescale for departure from flatness might be the Planck time, 10−43 seconds, the fact that the Universe has reached neither a heat death nor a Big Crunch after billions of years requires an explanation.
For instance, even at the relatively late age of a few minutes (the time of nucleosynthesis), the Universe density must have been within one part in 1014 of its critical value, or it would not exist as it does today.
For instance, even at the relatively late age of a few minutes (the time of nucleosynthesis), the Universe density must have been within one part in 1014 of its critical value, or it would not exist as it does today.
C ) - The magnetic monopole problem.
The magnetic monopole objection was raised in the late 1970s. Grand unification theories predicted topological defects in space that would manifest as magnetic monopoles. These objects would be produced efficiently in the hot early Universe, resulting in a density much higher than is consistent with observations, given that searches have never found any monopoles. This problem is also resolved by cosmic inflation, which removes all point defects from the observable Universe in the same way that it drives the geometry to flatness.
Big Bang Theory - What About God?
Any discussion of the Big Bang theory would be incomplete without asking the question, what about God? This is because cosmogony (the study of the origin of the universe) is an area where science and theology meet. Creation was a supernatural event.
That is, it took place outside of the natural realm. This fact begs the question: is there anything else which exists outside of the natural realm? Specifically, is there a master Architect out there? We know that this universe had a beginning. Was God the "First Cause"? We won't attempt to answer that question in this short article.
VSL - Varying Speed of Light
João Magueijo studied physics at the University of Lisbon. He undertook graduate work and Ph.D. at Cambridge University. He was awarded a research fellowship at St John's College, Cambridge, the same fellowship previously held by Paul Dirac and Abdus Salam. He has been a faculty member at Princeton and Cambridge, and is currently a professor at Imperial College London where he teaches undergraduates "General Relativity" and postgraduates "Advanced General Relativity".
In 1998, Magueijo teamed with Andreas Albrecht to work on the varying speed of light (VSL) theory of cosmology, which proposes that the speed of light was much higher in the early universe, of 60 orders of magnitude faster than its present value. This would explain the horizon problem (since distant regions of the expanding universe would have had time to interact and homogenize their properties), and is presented as an alternative to the more mainstream theory of cosmic inflation.
Magueijo discusses his personal struggles pursuing VSL in his 2003 book, Faster Than The Speed of Light, The Story of a Scientific Speculation. He was also associated with a dispute over priority concerning VSL with John Moffat. He was also the host of the Science Channel special, João Magueijo's Big Bang, which premiered on May 13, 2008.
In 2009, he published A Brilliant Darkness, an account of the life and science of vanished physicist Ettore Majorana.
ALICE (Informação do CERN)
ALICE (A Large Ion Collider Experiment) é um detector de íons pesados instalados no anel do Grande Colisor de Hádrons (LHC). Foi concebido para estudar as propriedades físicas do material submetido a uma forte interacção, em que uma fase de matéria chamado quark-glúon é formada de densidades de energia extremas. ALICE estuda o plasma de quarks e glúons, um estado da matéria que existia logo após o Big Bang.
Toda a matéria comum presente no Universo hoje é composta de átomos . Cada átomo é formado por um núcleo , composto de protões e neutrões (com excepção de hidrogénio , que não tem de neutrões ) e rodeado por uma nuvem de electrões . Protões e neutrões , por sua vez , são compostos de quarks unidos por outras partículas chamadas gluões . Um quark nunca foi observado isoladamente: quarks e gluões parecem estar inter-relacionados e confinados permanentemente para partículas compostas , tais como protões e neutrões. Este fenómeno é conhecido como contenção .
As colisões que ocorrem no LHC geram temperaturas mais de 100 mil vezes mais altas do que as praticadas no centro do sol. Todos os anos, durante certos períodos , o LHC faz colidir íons de chumbo para recriar em laboratório, condições semelhantes às que existiram logo após as condições do Big Bang .
As colisões que ocorrem no LHC geram temperaturas mais de 100 mil vezes mais altas do que as praticadas no centro do sol. Todos os anos, durante certos períodos , o LHC faz colidir íons de chumbo para recriar em laboratório, condições semelhantes às que existiram logo após as condições do Big Bang .
Nessas condições extremas, os protões e neutrões "derretem", liberando a ligação de quarks e gluão que formam o plasma quark-gluão. A existência desta fase e em particular as suas propriedades, são na teoria da cromodinâmica quântica (QCD), os elementos-chave para a compreensão do confinamento e o problema físico chamado restauração da simetria quiral. A colaboração ALICE estudou o plasma quark-gluão como ele se expande e se resfria, observando como ele progressivamente dá origem a partículas que constituem a matéria do nosso universo.
Para estudar o plasma quark-gluão, a colaboração ALICE usa o detector ALICE, que pesa 10.000 toneladas e tem 26 metros de comprimento, 16 metros de altura e 16 metros de largura. Está situado em uma vasta caverna localizada 56 metros de profundidade perto da aldeia de Saint-Genis-Pouilly (França), onde recebe os feixes no LHC.
Colaboração de mais de 1.000 cientistas de mais de 100 instituições de 30 países.
Nuclear Binding Energy
Nuclei are made up of protons and neutron, but the mass of a nucleus is always less than the sum of the individual masses of the protons and neutrons which constitute it. The difference is a measure of the nuclear binding energy which holds the nucleus together. This binding energy can be calculated from the Einstein relationship:
For the alpha particle Δm= 0.0304 u which gives a binding energy of 28.3 MeV.
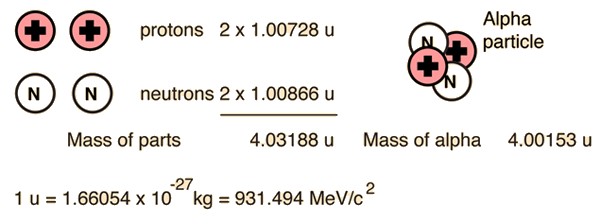
The enormity of the nuclear binding energy can perhaps be better appreciated by comparing it to the binding energy of an electron in an atom. The comparison of the alpha particle binding energy with the binding energy of the electron in a hydrogen atom is shown below. The nuclear binding energies are on the order of a million times greater than the electron binding energies of atoms.
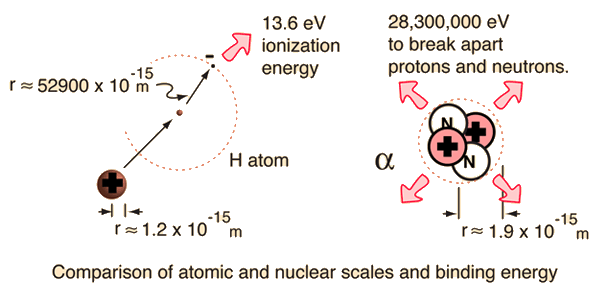
Fission and fusion can yield energy
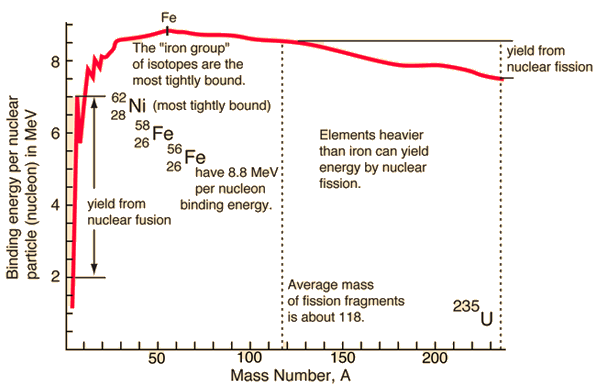
Nuclear Binding Energy Curve
The binding energy curve is obtained by dividing the total nuclear binding energy by the number of nucleons. The fact that there is a peak in the binding energy curve in the region of stability near iron means that either the breakup of heavier nuclei (fission) or the combining of lighter nuclei (fusion) will yield nuclei which are more tightly bound (less mass per nucleon).
The buildup of heavier elements in the nuclear fusion processes in stars is limited to elements below iron, since the fusion of iron would subtract energy rather than provide it. Iron-56 is abundant in stellar processes, and with a binding energy per nucleon of 8.8 MeV, it is the third most tightly bound of the nuclides. Its average binding energy per nucleon is exceeded only by 58Fe and 62Ni, the nickel isotope being the most tightly bound of the nuclides.
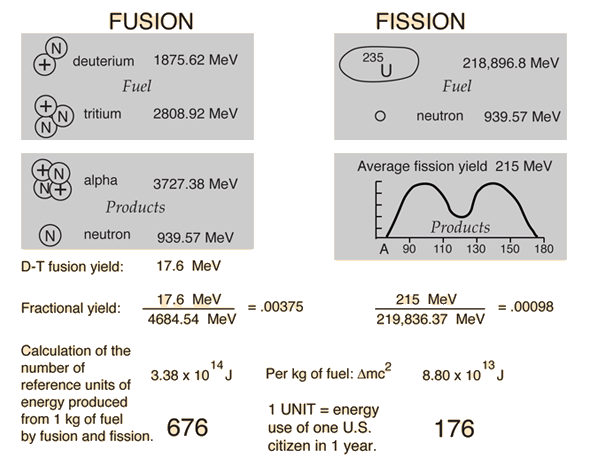
Deuterium-tritium fusion and uranium-235 fission are compared in terms of energy yield. Both the single event energy and the energy per kilogram of fuel are compared. Then they expressed in terms of a nominal per capita U.S. energy use: 5 x 1011 joules. This figure is dated and probably high, but it gives a basis for comparison. The values above are the total energy yield, not the energy delivered to a consumer.
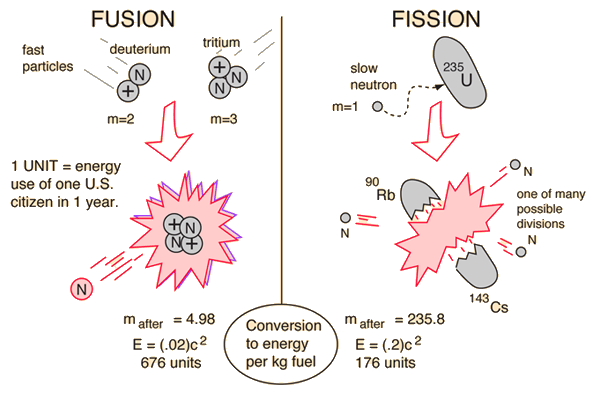
Conservation of Energy
The relativistic energy expression E = mc2 is a statement about the energy an object contains as a result of its mass and is not to be construed as an exception to the principle of conservation of energy. Energy can exist in many forms, and mass energy can be considered to be one of those forms. "Energy is the ultimate convertable c
Pair Production
Every known particle has an antiparticle; if they encounter one another, they will annihilate with the production of two gamma-rays. The quantum energies of the gamma rays is equal to the sum of the mass energies of the two particles (including their kinetic energies). It is also possible for a photon to give up its quantum energy to the formation of a particle-antiparticle pair in its interaction with matter.
The rest mass energy of an electron is 0.511 MeV, so the threshold for electron-positron pair production is 1.02 MeV. For x-ray and gamma-ray energies well above 1 MeV, this pair production becomes one of the most important kinds of interactions with matter. At even higher energies, many types of particle-antiparticle pairs are produced.
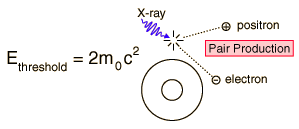
Relativistic Kinetic Energy
The relativistic energy expression includes both rest mass energy and the kinetic energy of motion. The kinetic energy is then given by
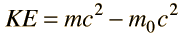
This is essentially defining the kinetic energy of a particle as the excess of the particle energy over its rest mass energy. For low velocities this expression approaches the non-relativistic kinetic energy expression.
![]() |
The relativistic energy expression includes both rest mass energy and the kinetic energy of motion. The kinetic energy is then given by
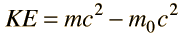
![]() |
The relativistic kinetic energy expression can be written as
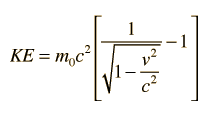
and the square root expression then expanded by use of the binomial theorem :

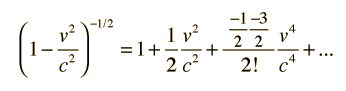
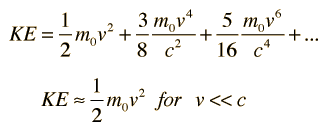
Dark matter
During the 1970s and 1980s, various observations showed that there is not sufficient visible matter in the Universe to account for the apparent strength of gravitational forces within and between galaxies.
This led to the idea that up to 90% of the matter in the Universe is dark matter that does not emit light or interact with normal baryonic matter. In addition, the assumption that the Universe is mostly normal matter led to predictions that were strongly inconsistent with observations.
In particular, the Universe today is far more lumpy and contains far less deuterium than can be accounted for without dark matter. While dark matter has always been controversial, it is inferred by various observations: the anisotropies in the CMB, galaxy cluster velocity dispersions, large-scale structure distributions, gravitational lensing studies, and X-ray measurements of galaxy clusters.
Indirect evidence for dark matter comes from its gravitational influence on other matter, as no dark matter particles have been observed in laboratories. Many particle physics candidates for dark matter have been proposed, and several projects to detect them directly are underway.
Steady State theory
In cosmology, the Steady State theory is a now-obsolete theory and model alternative to the Big Bang theory of the universe's origin (the standard cosmological model). In steady state views, new matter is continuously created as the universe expands, thus adhering toperfect cosmological principle.
While the steady state model enjoyed some popularity in the first half of the 20th century, it is now rejected by the vast majority of professional cosmologists and other scientists, as the observational evidence points to a Big Bang-type cosmology and a finite age of the universe.
Sir James Jeans, in the 1920s, was the first to conjecture a steady state cosmology based on a hypothesized continuous creation of matter in the universe. The idea was then revised in 1948 by Fred Hoyle, Thomas Gold, Hermann Bondi and others. The steady state theory of Bondi and Gold was inspired by the circular plot of the film Dead of Night, which they had watched together. Theoretical calculations showed that a static universe was impossible under general relativity, and observations by Edwin Hubble had shown that the universe was expanding. The steady state theory asserts that although the universe is expanding, it nevertheless does not change its appearance over time (the perfect cosmological principle); it has no beginning and no end.
Problems with the steady-state theory began to emerge in the late 1960s, when observations apparently supported the idea that the universe was in fact changing: quasars and radio galaxies were found only at large distances (therefore existing only in the distant past), not in closer galaxies. Whereas the Big Bang theory predicted as much, the Steady State theory predicted that such objects would be found everywhere, including close to our own galaxy.
For most cosmologists, the refutation of the steady-state theory came with the discovery of the cosmic microwave background radiation in 1965, which was predicted by the Big Bang theory. Stephen Hawking said that the fact that microwave radiation had been found, and that it was thought to be left over from the Big Bang, was "the final nail in the coffin of the steady-state theory". Within the steady state theory this background radiation is the result of light from ancient stars which has been scattered by galactic dust.
However, this explanation has been unconvincing to most cosmologists as the cosmic microwave background is very smooth, making it difficult to explain how it arose from point sources, and the microwave background shows no evidence of features such as polarization which are normally associated with scattering. Furthermore, its spectrum is so close to that of an ideal black body that it could hardly be formed by the superposition of contributions from dust clumps at different temperatures as well as at different redshifts.Steven Weinberg wrote in 1972,
- The steady state model does not appear to agree with the observed dL versus z relation or with source counts ... In a sense, the disagreement is a credit to the model; alone among all cosmologies, the steady state model makes such definite predictions that it can be disproved even with the limited observational evidence at our disposal. The steady-state model is so attractive that many of its adherents still retain hope that the evidence against it will disappear as observations improve. However, if the cosmic microwave background radiation ... is really black-body radiation, it will be difficult to doubt that the universe has evolved from a hotter, denser early stage.
Since that time, the Big Bang theory has been considered to be the best description of the origin of the universe. In most astrophysical publications, the Big Bang is implicitly accepted and is used as the basis of more complete theories.
Other proponents
Chaotic Inflation theory has many similarities with steady state theory, however on a much larger scale than originally envisaged. It is the C-field and the notion of quasi-steady state universe that has some resemblance to chaotic inflation theory or eternal inflation, which sometimes posits an infinite universe with neither beginning nor end, in which inflation operates continuously, on a scale beyond the observable universe, to create the matter of the cosmos. However, both steady state and quasi-steady state assert that the creation events of the universe (new hydrogen atoms in the steady state case) can be observed within the observable universe, whereas inflationary theories do not posit inflation as an ongoing process within the observable universe.