Uranium Bomb
Using the energy release from the nuclear fission of uranium-235, an explosive device can be made by simply positioning two masses of U-235 so that they can be forced together quickly enough to form a critical mass and a rapid, uncontrolled fission chain reaction. That is not to say that this is an easy task to accomplish. First you must obtain enough uranium which is highly enriched to over 90% U-235, whereas natural uranium is only 0.7% U-235.
This enrichment is an exceptionally difficult task, a fact that has helped control the proliferation of nuclear weapons. Once the required mass is obtained, it must be kept in two or more pieces until the moment of detonation. Then the pieces must be forced together quickly and in such a geometry that the generation time for fission is extremely short.
This leads to an almost instantaneous buildup of the chain reaction, creating a powerful explosion before the pieces can fly apart. Two hemispheres which are explosively forced into contact can produce a bomb such as the one detonated at Hiroshima.
Plutonium Bomb
Plutonium-239 is a fissionable isotope and can be used to make a nuclear fission bomb similar to that produced with uranium-235. The bomb which was dropped at Nagasaki was a plutonium bomb. Not enough Pu-239 exists in nature to make a major weapons supply, but it is easily produced in breeder reactors. In the U.S., there are reactors at Savannah River Plant, S.C., and at Hanford, Washington which are classified as plutonium production reactors.
They breed plutonium by surrounding a fission reactor with a uranium-238 "blanket" to make use of the breeding reaction between neutrons and U-238. Once the plutonium is produced, it is easily separated from the other fission products by chemical means, so that less technology is needed to produce a nuclear weapon if you have a breeder reactor. This makes plutonium a greater source of concern for weapons proliferation, because reactors which appear to be just electric power generators can be breeding plutonium for weapons along with the power production.
The type of bomb which was dropped on Nagasaki on August 9, 1945 had been tested at Alamagordo, New Mexico on July 16. It developed from the Manhattan Project after Fermi demonstrated in 1942 that a sustained nuclear chain reaction was possible.
Hiroshima
On August 6, 1945, a uranium fission bomb was detonated over the Japanese city of Hiroshima. The bomb, called "Little Boy" was a "gun-type" device which used an explosive charge to force two sub-critical masses of U-235 together. It was 28 inches in diameter and 120 inches long, a relatively small package to deliver an explosive force of some 20,000 tons of TNT by converting about 1 gram of matter into energy.
This could be accomplished with a sphere of U-235 about the size of a baseball. This kind of device had never been tested, in contrast to the plutonium bomb which was dropped on Nagasaki three days later. No device like this has been used since, making the estimates of radiation exposure at Hiroshima very difficult. Casualties included both direct blast victims plus those who died from radiation-induced cancer in subsequent years.
The bomb was triggered to explode at a height of 550 meters (1800 ft), a height calculated to cause the widest area of damage. In the detonation of the uranium fission bomb over Hiroshima, about 130,000 people were reported killed, injured, or missing. Another 177,000 were
Nagasaki
On August 9, 1945 a plutonium fission bomb was detonated over the Japanese city of Nagasaki, three days after a uranium fission bomb was dropped on Hiroshima. The bomb, called "Fat Man", was 128 inches long and had a diameter of 60.5 inches. It used implosion to compress the sub-critical assembly of plutonium. This kind of device had been tested less than a month before the drop, and was the subject of several other weapons tests after World War II. The explosive yield was about 20,000 tons of TNT, generated in about a microsecond.
The bomb was triggered to explode at a height of 550 meters (1800 ft), a height calculated to cause the widest area of damage.
Thermonuclear Explosions
Because of the high temperatures required to initiate a nuclear fusion reaction, such devices are often called thermonuclear devices. A thermonuclear explosion can be created only by producing the required temperature, about a hundred-million Kelvins, and by forcing the material together so quickly that it will fuse rapidly. This is typically done with the isotopes of hydrogen, deuterium and tritium. This led to the term "hydrogen bomb" to describe the deuterium-tritium fusion bomb.
To obtain the two parts of the fuel, pellets were made from lithium hydride, LiD, made with the deuterium isotope. The only way which was found to produce the ignition temperature was to set off a fission bomb such that it would heat and compress the lithium hydride. In the process, the lithium was bombarded with neutrons, breeding tritium. Then the deuterium-tritium fusion reaction could take place.
Hydrogen Bomb
Because the thermonuclear explosive devices used hydrogen isotopes, (deuterium-tritium fusion), the resulting bombs were often called "hydrogen bombs". The first hydrogen bomb was detonated on November 1, 1952 at the small island Eniwetok in the Marshall Islands. It's yield was several megatons of TNT. The Soviet Union detonated a fusion bomb in the megaton range in August of 1953.
The U.S. exploded a 15 megaton fusion bomb on March 1, 1954. It had a fireball 4.8 km in diameter and created a huge characteristic mushroom-shaped cloud. Analysis of the radioactive fallout from this bomb revealed it to be a fission-fusion-fission weapon, a "hydrogen bomb" with an outer sheath of natural uranium to increase the yield
Tsar Bomba
"Tsar Bomb") is the nickname for the AN602 hydrogen bomb, the most powerful nuclear weapon ever detonated. Its October 30, 1961, test remains the most powerful artificial explosion in human history. It was also referred to as Kuz'kina Mat' (Russian:Кузькина мать, Kuzka's mother), referring to Nikita Khrushchev's promise to show the United States a "Kuz'kina Mat'" at the 1960 United Nations General Assembly.
The famous Russian idiom, which has been problematic for translators, literally meaning “to show somebody Kuzka's mother”, equates roughly with the English “We’ll show you!” Developed by the Soviet Union, the bomb had the yield of 50 to 58 megatons of TNT(210 to 240 PJ). Only one bomb of this type was ever officially built and it was tested on October 30, 1961, in the Novaya Zemlya archipelago, atSukhoy Nos.
The Tsar Bomba was a three-stage Teller–Ulam design hydrogen bomb with a yield of 50 to 58 megatons of TNT (210 to 240 PJ).[5] This is equivalent to about 1,350–1,570 times the combined power of the bombs that destroyed Hiroshima and Nagasaki,[6] 10 times the combined power of all the conventional explosives used in World War II, or one quarter of the estimated yield of the 1883 eruption of Krakatoa, and 10% of the combined yield of all nuclear tests to date.
A three-stage H-bomb uses a fission bomb primary to compress a thermonuclear secondary, as in most H-bombs, and then uses energy from the resulting explosion to compress a much larger additional thermonuclear stage. There is evidence that the Tsar Bomba had several third stages rather than a single very large one.
Nuclear Blast Damage
Blast damage from a nuclear weapon comes from the overpressure in the air and from winds which result from the pressures. For a 10 kiloton blast at the height where it would produce the most damage, severe damage to frame houses would occur out to 1.6 km and moderate damage to 2.4 km. For a 10 megaton blast, 1000 times as powerful, the severe damage would extend out about ten times as far, to 17.7 km. (Figures from Microsoft Encarta).
A 10 kiloton blast would produce a fireball of about 300 m diameter and would cause moderate flash burns (second degree) at a range of about 2.4 km. A 10 megaton blash would create a fireball about 4.8 km and moderate flash burns to 32 km. Accompanying the blast is a burst of neutrons and gamma rays, as well as lingering residual radiation from radioactive fallout.
Radioactive Fallout
In addition to the great destructive power of fission bombs, the highly radioactive fission byproducts are released into the atmosphere and spread over a wide area. Radioactive fallout in the form of fine particulate matter is particularly dangerous because it can be ingested, bringing alpha and beta emitters into the body where they can do much more damage. One of the most serious components of the fallout from weapons testing in the deserts of Arizona and Utah was strontium-90.
A Ton of TNT
For some reason, the comparison unit for nuclear explosions which became most popular was the "ton of TNT". A nominal energy release for a ton of TNT can be extracted from general statements about nuclear weapons. One of those is "one kilogram of mass converted to energy is equivalent to about 22 megatons of TNT". From the Einstein equation, the conversion is
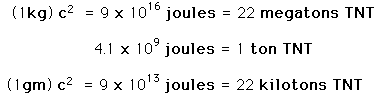
This is consistent with the oft-quoted statement that the 20 kiloton Hiroshima bomb converted about 1 gram of mass to energy.
Nuclear Winter
Riddle: What has a dust storm on Mars have to do with the nuclear arms race?
Answer: A massive dust storm on Mars, observed by the Mariner 9 spacecraft, obscured the surface for weeks and dramatically lowered the surface temperature under the dust cloud.
This was perhaps the first clear evidence that a major nuclear weapon exchange could have catastrophic global climatic effects. Air bursts tend to blow fine particulate matter high into the atmosphere where it could stay for months or years. The term "Nuclear Winter" was introduced by the TTAPS group to describe the cold and dark which would accompany this high particulate cloud.
Fission Fragments
![]() |
When uranium-235 undergoes fission, the average of the fragment mass is about 118, but very few fragments near that average are found. It is much more probable to break up into unequal fragments, and the most probable fragment masses are around mass 95 and 137.
Most of these fission fragments are highly unstable (radioactive), and some of them such as cesium-137 and strontium-90 are extremely dangerous when released to the environment.
|
Fission Fragment Example
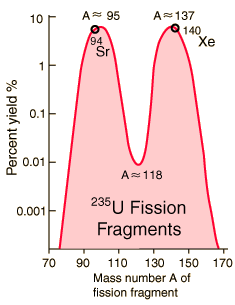
A common pair of fragments from uranium-235 fission is xenon and strontium:

Highly radioactive, the xenon decays with a half-life of 14 seconds and finally produces the stable isotope cerium-140. Strontium-94 decays with a half-life of 75 seconds, finally producing the stable isotope zirconium-94. These fragments are not so dangerous as intermediate half-life fragments such as cesium-137.
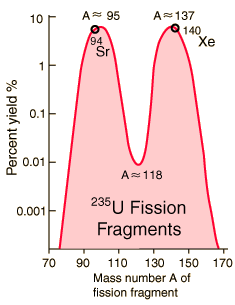
A common pair of fragments from uranium-235 fission is xenon and strontium:

Highly radioactive, the xenon decays with a half-life of 14 seconds and finally produces the stable isotope cerium-140. Strontium-94 decays with a half-life of 75 seconds, finally producing the stable isotope zirconium-94. These fragments are not so dangerous as intermediate half-life fragments such as cesium-137.
Fission Fragment Decay
This particular set of fragments from uranium-235 fission undergoes a series of beta decays to form stable end products.
![]()
Cesium-137
Cesium-137 and strontium-90 are the most dangerous radioisotopes to the environment in terms of their long-term effects. Their intermediate half-lives of about 30 years suggests that they are not only highly radioactive but that they have a long enough halflife to be around for hundreds of years. Iodine-131may give a higher initial dose, but its short halflife of 8 days ensures that it will soon be gone.
Besides its persistence and high activity, cesium-137 has the further insidious property of being mistaken for potassium by living organisms and taken up as part of the fluid electrolytes. This means that it is passed on up the food chain and reconcentrated from the environment by that process.
Strontium-90
Strontium-90 and cesium-137 are the radioisotopes which should be most closely gaurded against release into the environment. They both have intermediate halflives of around 30 years, which is the worst range for half-lives of radioactive contaminants. It ensures that they are not only highly radioactive but also have a long enough halflife to be around for hundreds of years.
Strontium-90 mimics the properties of calcium and is taken up by living organisms and made a part of their electrolytes as well as deposited in bones. As a part of the bones, it is not subsequently excreted like cesium-137 would be. It has the potential for causing cancer or damaging the rapidly reproducing bone marrow cells.
Strontium-90 is not quite as likely as cesium-137 to be released as a part of a nuclear reactor accident because it is much less volatile, but is probably the most dangerous component of the radioactive fallout from a nuclear weapon.
Strontium-90 undergoes beta decay, emitting electrons with energy 0.546 MeV with a half-life of 28.8 years. The decay product is yttrium-90.
Iodine-131
Iodine-131 is a major concern in any kind of radiation release from a nuclear accident because it is volatile and because it is highly radioactive, having an 8 day half-life. It is of further concern in the human body because iodine is quickly swept up by the thyroid, so that the total intake of iodine becomes concentrated there.
The thyroid has a maximum uptake of iodine, however, so some protection against iodine releases can be afforded by taking potassium iodide tablets to load up the thyroid to capacity so that radioactive iodine would be more likely to be excreted.
Implosion-Type Bomb![]() Implosion Detonation![]() Implosion DetonationOne of the methods for quickly producing a super-critical mass of plutonium for a nuclear explosion is to fashion a sub-critical mass of Pu-239 in a spherical shape and then set off high explosives to drive it inward. A spherical shell of high-explosive material is made up of fitting pieces (called "lenses" to focus the explosion inward) which are wired with detonators to set them off simultaneously.This creates enough force to increase the density of the sphere of plutonium to the point where it is super-critical. This implosion technique was used in the first plutonium bomb test at Alamogordo and in the Nagasaki bomb, each with an explosive power of about 20 kilotons of TNT. The chain reaction lasts about a microsecond. Gun-Type Nuclear DetonationsWhen one sub-critical mass of a fissile material is held in place and another sub-critical mass is fired into it with the use of high explosives such that the assembly is then super-critical, this is called a gun-type nuclear device. This kind of device was used to construct the Hiroshima bomb. The explosive must force the additional mass into contact so that it maintains contact for about a microsecond, the time it takes for the rapid chain reaction to produce the nuclear explosion. The explosive mass is held in place partly by a heavy, solid surrounding shell called a tamper.![]() |
Uranium-235 Fission
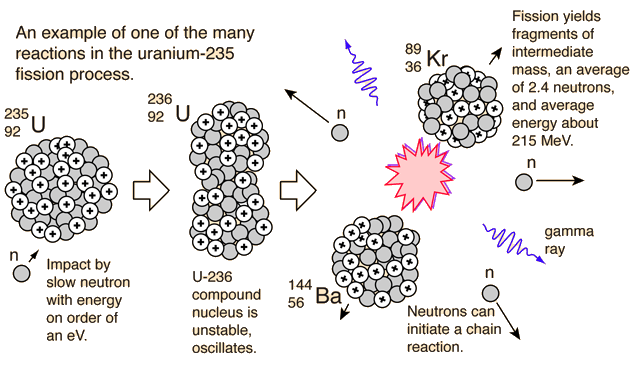
Uranium-235 Fission Example
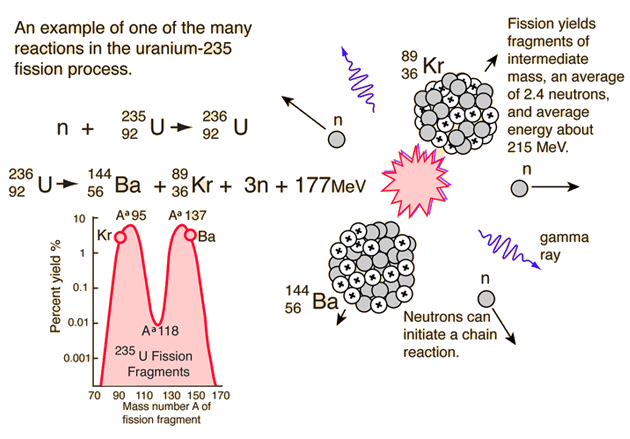
Energy From Uranium Fission
| Index Fission concepts Reference Mulligan Table 12.3. | ||||||||||||||||||||
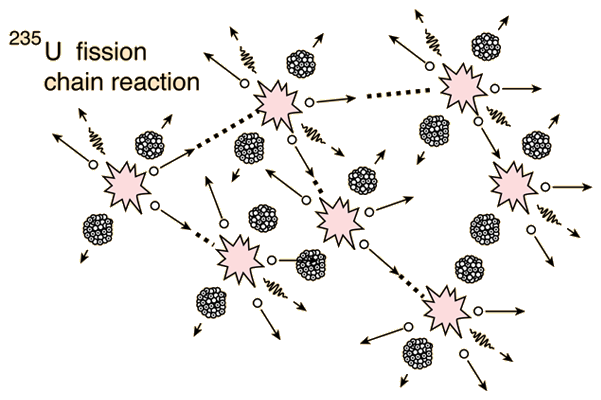
If at least one neutron from each fission strikes another U-235 nucleus and initiates fission, then the chain reaction is sustained.
Uranium-235 Chain Reaction
If an least one neutron from U-235 fission strikes another nucleus and causes it to fission, then the chain reaction will continue. If the reaction will sustain itself, it is said to be "critical", and the mass of U-235 required to produced the critical condition is said to be a "critical mass".
A critical chain reaction can be achieved at low concentrations of U-235 if the neutrons from fission are moderated to lower their speed, since the probability for fission with slow neutrons is greater.
| ![]()
A fission chain reaction produces intermediate mass fragments which are highly radioactiveand produce further energy by their radioactive decay. Some of them produce neutrons, called delayed neutrons, which contribute to the fission chain reaction.
|
Example of Energy Yield: Uranium-235 Fission
Nuclear fission of uranium-235 yields an enormous amount of energy from the fact that the fission products have less total mass than the uranium nucleus, a mass change that is converted to energy by the Einstein relationship E = mc2.
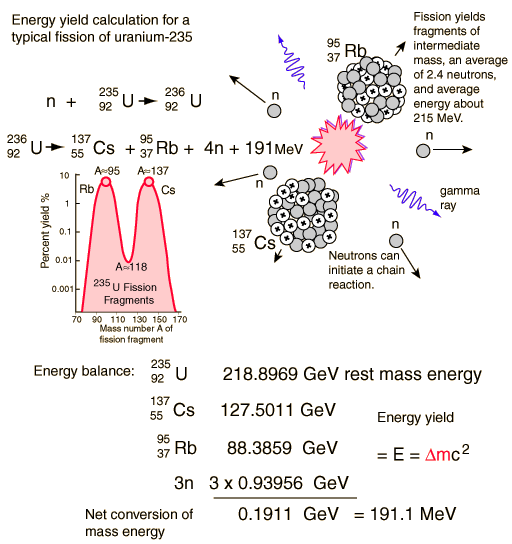
Sem comentários:
Enviar um comentário