You may click on any of the types of radiation for more detail about its particular type of interaction with matter. The different parts of theelectromagnetic spectrum have very different effects upon interaction with matter. Starting with low frequency radio waves, the human body is quite transparent. (You can listen to your portable radio inside your home since the waves pass freely through the walls of your house and even through the person beside you!) As you move upward through microwaves and infrared to visible light, you absorb more and more strongly. In the lower ultraviolet range, all the uv from the sun is absorbed in a thin outer layer of your skin.
As you move further up into the x-ray region of the spectrum, you become transparent again, because most of the mechanisms for absorption are gone. You then absorb only a small fraction of the radiation, but that absorption involves the more violent ionization events.
Each portion of the electromagnetic spectrum has quantum energies appropriate for the excitation of certain types of physical processes. The energy levels for all physical processes at the atomic and molecular levels are quantized, and if there are no available quantized energy levels with spacings which match the quantum energy of the incident radiation, then the material will be transparent to that radiation, and it will pass through.
Energy in Electromagnetic Waves
Electromagnetic waves carry energy as they travel through empty space. There is an energy density associated with both the electric and magnetic fields. The rate of energy transport per unit area is described by the vector
which is called the Poynting vector. This expression is a vector product, and since the magnetic field is perpendicular to the electric field, the magnitude can be written
The rate of energy transport S is perpendicular to both E and B and in the direction of propagation of the wave. A condition of the wave solution for a plane wave is Bm = Em/c so that the average intensity for a plane wave can be written
This makes use of the fact that the average of the square of a sinusoidal function over a whole number of periods is just 1/2.
How much power is transported by a string wave?
As a sinusoidal wave moves down a string, the energy associated with one wavelength on the string is transported down the string at the propagation velocity v. From the basic wave relationship, the distance traveled in one period is vT = λ, so the energy is transported one wavelength per period of the oscillation.
The energy associated with one wavelength of the wave is
so the transported power is | 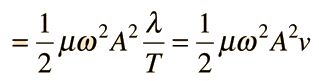 |
For a stretched string with mass per unit length m = grams/m
and tension T = Newtons
the propagation speed is v = m/s
For a wave of amplitude A = m
and angular frequency ω = radians/s
the transmitted power is P = watts.
Energy in a String Wave
The energy associated with a traveling wave in a stretched string is conveniently expressed as the energy per wavelength.
The energy of a small segment of the string can be expressed as the sum of the kinetic energy and elastic potential energy of the segment. The differential form of the elastic potential energy is
Using the description of a traveling wave
the potential energy expression becomes
The energy for a full wavelength can be found by integrating this expression at a given time, and it is most convenient to set t=0 for this integration. The energy for one wavelength along the string is
The differential kinetic energy is
Using the velocity expression
the kinetic energy takes the form
and again setting t=0 for simplification
The total energy associated with a wavelength is
Since this amount of energy is transported a distance of one wavelength along the string in one period, this expression can be used to calculate the power transmitted along a string.
Microwave Interactions
The quantum energy of microwave photons is in the range 0.00001 to 0.001 eV which is in the range of energies separating the quantum states of molecular rotation and torsion. The interaction of microwaves with matter other than metallic conductors will be to rotate molecules and produce heat as result of that molecular motion. Conductors will strongly absorb microwaves and any lower frequencies because they will cause electric currents which will heat the material.
Most matter, including the human body, is largely transparent to microwaves. High intensity microwaves, as in a microwave oven where they pass back and forth through the food millions of times, will heat the material by producing molecular rotations and torsions. Since the quantum energies are a million times lower than those of x-rays, they cannot produce ionization and the characteristic types of radiation damage associated with ionizing radiation.
Infrared Interactions
The quantum energy of infrared photons is in the range 0.001 to 1.7 eV which is in the range of energies separating the quantum states of molecular vibrations. Infrared is absorbed more strongly than microwaves, but less strongly than visible light. The result of infrared absorption is heating of the tissue since it increases molecular vibrational activity. Infrared radiation does penetrate the skin further than visible light and can thus be used for photographic imaging of subcutaneous blood vessels.
Visible Light Interactions
The primary mechanism for the absorption of visible light photons is the elevation of electrons to higher energy levels. There are many available states, so visible light is absorbed strongly. With a strong light source, red light can be transmitted through the hand or a fold of skin, showing that the red end of the spectrum is not absorbed as strongly as the violet end.
While exposure to visible light causes heating, it does not cause ionization with its risks. You may be heated by the sun through a car windshield, but you will not be sunburned - that is an effect of the higher frequency uv part of sunlight which is blocked by the glass of the windshield.
Ultraviolet Interactions
The near ultraviolet is absorbed very strongly in the surface layer of the skin by electron transitions. As you go to higher energies, the ionization energies for many molecules are reached and the more dangerous photoionization processes take place.
Sunburn is primarily an effect of uv, and ionization produces the risk of skin cancer. The ozone layer in the upper atmosphere is important for human health because it absorbs most of the harmful ultraviolet radiation from the sun before it reaches the surface.
The higher frequencies in the ultraviolet are ionizing radiation and can produce harmful physiological effects ranging from sunburn to skin cancer. Health concerns for UV exposure are mostly for the range 290-330 nm in wavelength, the range called UVB. According to Scotto, et al, the most effective biological wavelength for producing skin burns is 297 nm.
Their research indicates that the biological effects increase logarithmically within the UVB range, with 330 nm being only 0.1% as effective as 297 nm for biological effects. So it is clearly important to control exposure to UVB.
Broadcast Signals
Radio communication is typically in the form of AM radio or FM Radio transmissions. The broadcast of a single signal, such as a monophonic audio signal, can be done by straightforward amplitude modulation or frequency modulation.
More complex transmissions utilize sidebands arising from the sum and difference frequencies which are produced by superposition of some signal upon the carrier wave. For example, in FM stereo transmission, the sum of left and right channels (L+R) is used to frequency modulate the carrier and a separate subcarrier at 38 kHz is also superimposed on the carrier.
That subcarrier is then modulated with a (L-R) or difference signal so that the transmitted signal can be separated into left and right channels for stereo playback. In television transmission, three signals must be sent on the carrier: the audio, picture intensity, and picture chrominance. This process makes use of two subcarriers. Other transmissions such as satellite TV and long distance telephone transmission make use of multiple subcarriers for the broadcast of multiple signals simultaneously.
AM Radio
When information is broadcast from an AM radio station, the electrical image of the sound (taken from a microphone or other program source) is used to modulate the amplitude of the carrier wave transmitted from the broadcast antenna of the radio station. This is in contrast to FM radio where the signal is used to modulate the frequency of the carrier.
The AM band of the Electromagnetic spectrum is between 535 KHz and 1605 kHz and the carrier waves are separated by 10 kHz.
A radio receiver can be tuned to receive any one of a number of radio carrier frequencies in the area of the receiver. This is made practical by transferring the signal from the carrier onto an intermediate frequency in the radio by a process called heterodyning. In a heterodyne receiver, most of the electronics is kept tuned to the intermediate frequency so that only a small portion of the receiver circuit must be retuned when changing stations.
AM Radio
AM radio uses the electrical image of a sound source to modulate the amplitude of a carrier wave. At the receiver end in the detection process, that image is stripped back off the carrier and turned back into sound by a loudspeaker.
FM Radio
FM radio uses the electrical image of a sound source to modulate the frequency of a carrier wave. At the receiver end in the detection process, that image is stripped back off the carrier and turned back into sound by a loudspeaker.
FM Radio
When information is broadcast from an FM radio station, the electrical image of the sound (taken from a microphone or other program source) is used to modulate the frequency of the carrier wave transmitted from the broadcast antenna of the radio station. This is in contrast to AM radio where the signal is used to modulate the amplitude of the carrier.
The FM band of the electromagnetic spectrum is between 88 MHz and 108 MHz and the carrier waves for individual stations are separated by 200 kHz for a maximum of 100 stations. These FM stations have a 75 kHz maximum deviation from the center frequency, which leaves 25 kHz upper and lower "gaurd bands" to minimize interaction with the adjacent frequency band. This separation of the stations is much wider than that for AM stations, allowing the broadcast of a wider frequency band for higher fidelity music broadcast. It also permits the use of sub-carriers which make possible the broadcast of FM Stereo signals.
Frequency Modulation
The change in frequency, which is greatly exaggerated here, is proportional to the amplitude of the signal. An FM radio carrier around 100 MHz is limited to modulation of +/- 0.1 MHz. Normal FM stereo broadcast is within +/- .053 MHz.
AM and FM Radio Frequencies
The Amplitude Modulated (AM radio) carrier frequencies are in the frequency range 535-1605 kHz. Carrier frequencies of 540 to 1600 kHz are assigned at 10 kHz intervals.
The FM radio band is from 88 to 108 MHz between VHF television Channels 6 and 7. The FM stations are assigned center frequencies at 200 kHz separation starting at 88.1 MHz, for a maximum of 100 stations. These FM stations have a 75 kHz maximum deviation from the center frequency, which leaves 25 kHz upper and lower "gaurd bands" to minimize interaction with the adjacent frequency band.
FM Stereo Broadcast Band
The bandwidth assigned to each FM station is sufficently wide to broadcast high-fidelity, stereo signals. The carrier frequency is directly modulated with the sum of the left and right channel audio signals. A 38 kHz subcarrier also modulates the carrier, and that subcarrier is modulated with the difference, L- R , of the audio signals. The FM tuner then decodes this signal and separates the Left and Right audio channels.
Heterodyne Principle
Heterodyning is a method for transferring a broadcast signal from its carrier to a fixed local intermediate frequency in the receiver so that most of the receiver does not have to be retuned when you change channels. The interference of any two waves will produce a beat frequency, and this technique provides for the tuning of a radio by forcing it to produce a specific beat frequency called the "intermediate frequency" or IF.
An electromagnetic carrier wave which is carrying a signal by means of amplitude modulation or frequency modulation can transfer that signal to a carrier of different frequency by means of a process called heterodyning.This transfer is accomplished by mixing the original modulated carrier with a sine wave of another frequency. This process produces a beat frequencyequal to the difference between the frequencies, and this difference frequency constitutes a third carrier which will be modulated by the original signal.
Heterodyning is extremely important in radio transmission -- in fact, the development of heterodyning schemes was one of the major developments which led to mass communication by radio. By fixing the beat frequency between the incoming carrier and the local oscillator to a fixed intermediate frequency (IF), most of a radio receiver can be constructed so that it can be used by any incoming radio signal. Only the local oscillator is tuned to produce a beat frequency equal to the fixed IF frequency. We now take for granted that one radio receiver can be tuned to any of the locally broadcast radio stations, but if it were not for heterodyning, you would have to have one receiver for each broadcast station.
Sem comentários:
Enviar um comentário